Have you ever wondered how a deeper understanding of the body's energy systems could enhance your training and elevate your game performance? Many athletes focus on perfecting their routines, nutrition, and recovery, but can often overlook the crucial mechanisms that power their every move. The human body is an intricate machine, and its ability to generate and sustain energy efficiently is key to reaching peak performance. By exploring the science behind our energy systems, we can unlock the potential to train smarter and achieve superior results.
Energy Systems and Performance
Energy systems refer to the specific mechanisms by which your body produces and uses energy. You generate energy through three systems: the phosphagen (ATP-PC) system, the glycolytic system, and the oxidative system. All three energy systems are active during any form of physical activity.
The phosphagen system (ATP-PC system) relies on adenosine triphosphate (ATP) and phosphocreatine (PC) as its primary energy sources. It provides immediate energy for short, explosive movements such as sprinting, lifting heavy weights, or jumping, but is depleted quickly, typically within 10 seconds.
The glycolytic system uses glycogen stored in muscles and glucose in the blood as its primary energy sources. It supports high-intensity activities like sprints or high-intensity interval training (HIIT), producing energy more slowly than the phosphagen system. This system can sustain around two minutes but can lead to the buildup of lactate, causing muscle fatigue.
The oxidative system, which utilizes carbohydrates, fats, and, to a lesser extent, proteins, is the primary energy source for long-duration, low-to-moderate intensity activities such as distance running, cycling, or swimming. Although it produces energy at a slower rate, it can sustain energy production for longer periods, making it highly efficient for endurance activities.
System | Power (Rate of ATP Production) | Capacity (Total Ability to Produce ATP) | Fuel Used |
Phosphagen | Very High | Very Low | Creatine Phosphate Stored ATP |
Glycolytic | High | Low | Blood Glucose, Muscle and Liver Glycogen |
Oxidative | Low | Very High | Blood Glucose, Muscle and Liver Glycogen & Adipose, Intramuscular Fat |
During physical activity, these systems do not work in isolation but rather contribute concurrently to energy production, with the dominant system varying based on the specific demands of the activity. For instance, a sprinter primarily uses the phosphagen system but will also tap into the glycolytic and oxidative systems as the race progresses. Conversely, a marathon runner relies predominantly on the oxidative system but also uses the other systems at the start and during bursts of speed.
The Model of Physical Performance
Understanding the model of physical performance involves examining how several factors contribute to an athlete's ability to perform. This model can be broken down into three key components: speed of movement (physical), level of skill (technical), and strategy (tactical). Speed movement encompasses the raw physical abilities of the athlete, such as their maximum sprinting speed or the explosiveness of their movements. The level of skill refers to the technical skills an athlete possesses, such as their ability to execute precise movements or techniques required for their sport. Strategy involves the tactical decisions an athlete makes in competition, such as positioning, timing, and decision-making.
Several critical questions arise when considering physical performance: why do we fatigue, what determines an athlete's maximum speed, what determines an athlete's average or maximum work rate, how long can an athlete maintain speed and/or power, and how are speed, power, and fatigue all related? Athletic performance relies on different energy systems, each with distinct characteristics. The aerobic system depends on oxygen and produces the most ATP, though it takes longer and involves more steps. It sustains moderate outputs for very long durations, making it ideal for endurance activities. The anaerobic system regenerates ATP without the use of oxygen, doing so at a faster rate than the aerobic system but only for short durations, providing maximum power output for short bursts of activity.
Understanding the trade-off between force/power and endurance is crucial. Force and power, which relies on anaerobic energy, are characterized by fast-twitch, glycolytic, and alactic muscle fibers. These fibers have a large cross-sectional area and can generate maximum force but have low mitochondrial and capillary density. This system is optimal for the CNS, firing at a high rate. In contrast, endurance relies on aerobic energy and is characterized by slow-twitch, fatigue-resistant muscle fibers. These fibers support maximum oxygen transportation and have high mitochondrial density and oxidative enzyme activity, leading to improved “fuel economy” and a high VO2 max. By grasping these components and systems, athletes and coaches can better design training programs to optimize performance, balance the trade-offs between power and endurance, and address the factors that contribute to fatigue.
The Role of the Brain
The brain acts as a safety mechanism, slowing the athlete down to prevent injury or damage, which we experience as "fatigue." Fatigue is a complex phenomenon influenced by various physiological factors, including the brain's role in both the central nervous system (CNS) and the peripheral nervous system (PNS).
From a CNS take, fatigue involves a reduction in central drive, where the brain reduces the signals sent to the muscles, leading to a decrease in muscle activation and resulting in lower force and power output. This mechanism acts as a protective measure to prevent muscle damage and overexertion. The CNS also selectively recruits fewer motor units during prolonged or intense activity, helping manage energy reserves and preventing the complete depletion of resources. Additionally, the brain alters the athlete's behavior, often making them feel more fatigued or less motivated to continue at high intensity. Psychological factors, such as motivation and mental fatigue, contribute to this aspect of central fatigue.
From the PNS perspective, fatigue involves changes in the contractile properties of muscle fibers, such as reduced calcium release and impaired cross-bridge cycling, leading to decreased force production and slower muscle contraction velocity. The transmission of signals from nerves to muscles at the neuromuscular junction can become less efficient during prolonged activity, resulting in weaker muscle contractions and delayed responses. Changes in muscle metabolism, including a shift from aerobic to anaerobic energy production, can lead to fatigue, producing less efficient energy (ATP) and generating fatigue-inducing byproducts.
The brain's role in managing fatigue can be seen as a comprehensive safety mechanism, integrating both central and peripheral signals to protect the body from overexertion. The CNS receives feedback from peripheral sensors, such as muscle spindles and metabolic receptors, and adjusts the central drive to prevent damage. The brain interprets various physiological signals and modulates the perception of effort, influencing an athlete's willingness to continue at high intensity. By regulating both central and peripheral factors, the brain ensures that energy resources are used efficiently, balancing short-term performance with long-term health and recovery. Understanding the dual role of the brain in fatigue from both CNS and PNS perspectives highlights the importance of a holistic approach to training and recovery. By addressing both central and peripheral aspects of fatigue, athletes can optimize their performance and maintain their physical and mental well-being.
Conclusion
So, are you starting to see how speed, power, and fatigue are all connected? The phosphagen, glycolytic, and oxidative systems each play a unique role in producing energy for all physical activities. Additionally, the brain's role in managing fatigue highlights the importance of both central and peripheral factors in athletic performance. Knowing how the brain controls effort and energy use provides valuable insights for both the player and coach. By applying these insights, coaches and athletes alike can develop balanced training plans that maximize their potential while protecting their health and recovery. Ultimately, understanding energy systems, physical performance models, and how the brain's regulation helps athletes push their limits, achieve their goals, and maintain long-term well-being.
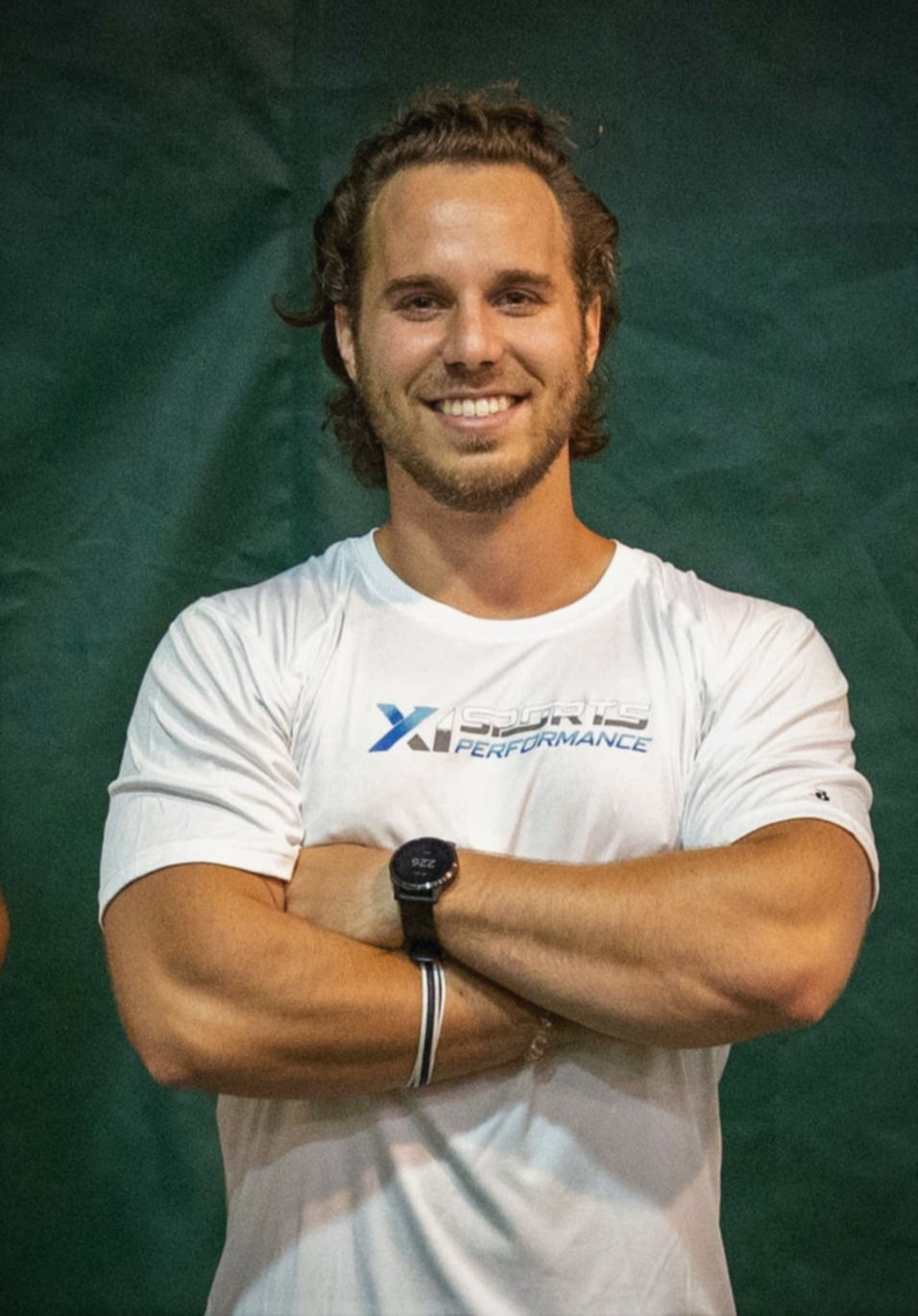
About the Author:
Nathan Albright is the owner of XI Sports Performance, where he works with youth, high school, and college-level athletes offering a wide variety of training with various sports, including Soccer, Football, Basketball, Baseball, Volleyball, and more. Nathan has a BSc. in Exercise Kinesiology from the University of Toledo, and currently resides in Northwest Ohio.
Comments